Crystal clear
Research reveals fundamental mechanism of colloid crystal growth, opens door to new applications
By Leah Burrows
August 19, 2021
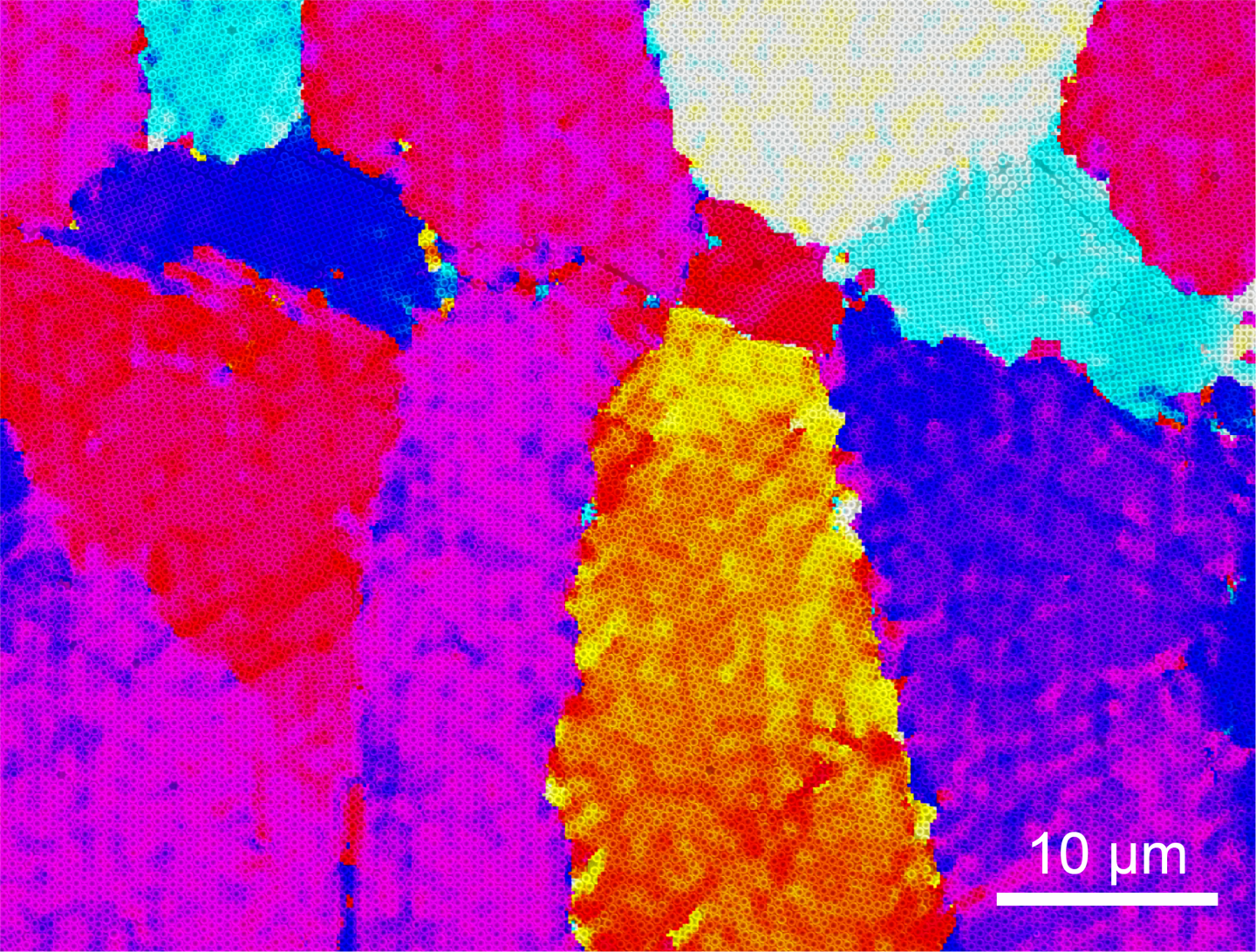
Colloidal particles—nano- to micrometer-sized particles suspended in liquid—will self-assemble into highly ordered crystal lattices without any external guidance through the simple process of evaporation. While these types of crystals, known as colloidal crystals, have been studied for decades, much is still unknown about the self-assembly process, including why these crystals often grow in a specific direction. Answering that question could help researchers design and engineer colloidal crystals for large-scale applications in optics, sensing and catalysis.
In a new paper, an interdisciplinary team of researchers used theory, modeling and experimentation to develop a comprehensive understanding of a fundamental mechanism underlying colloidal crystal growth. The researchers from the Harvard John A. Paulson School of Engineering and Applied Sciences (SEAS) are part of the National Science Foundation-supported Harvard Materials Research Science and Engineering Center (MRSEC).
"Self-assembly of colloidal particles is a widely used process, but little is known about the origin of the directional order in the resulting crystals. Our comprehensive research sheds new light on why colloidal crystals grow along a specific orientation and lays the foundation to control this growth for future applications," said Joanna Aizenberg, the Amy Smith Berylson Professor of Materials Science and Professor of Chemistry & Chemical Biology at SEAS and corresponding author of the study.
The research was published recently in the Proceedings of the National Academy of Science (PNAS).
Colloidal crystals grow through a process of evaporation similar to how coffee rings form. As the water in coffee evaporates, the coffee particles suspended in the liquid attach to a surface – such as a coffee table or coaster. In the case of colloidal crystals, as the liquid evaporates, the particles attach to the substrate and eventually assemble in crystals that are oriented in a specific direction. To understand how that happens, the researchers recorded the early stages of the assembly process using high-resolution microscopy and an algorithm to track the positions of individual nanoparticles. These techniques allowed the researchers to map and calculate the orientation of each colloidal particle during self-assembly.
The researchers found that at the very beginning of the process, the particles tend to align with the liquid-air interface as the liquid evaporates, despite some misoriented regions. Then, about 100 microns below where the liquid first started evaporating, the researchers observed a switch in which the particles rotated 90 degrees and began growing into a stable crystal lattice.
"With high-resolution imaging, we were able to observe the transition from the initial misoriented colloidal crystal to a highly ordered crystal with a specific crystal orientation aligned with the growth direction," said Ling Li, the co-corresponding author of the paper who started this work as a postdoctoral fellow at SEAS and continued as an Assistant Professor at Virginia Tech. "The next step was to understand how and why that rotation takes place."
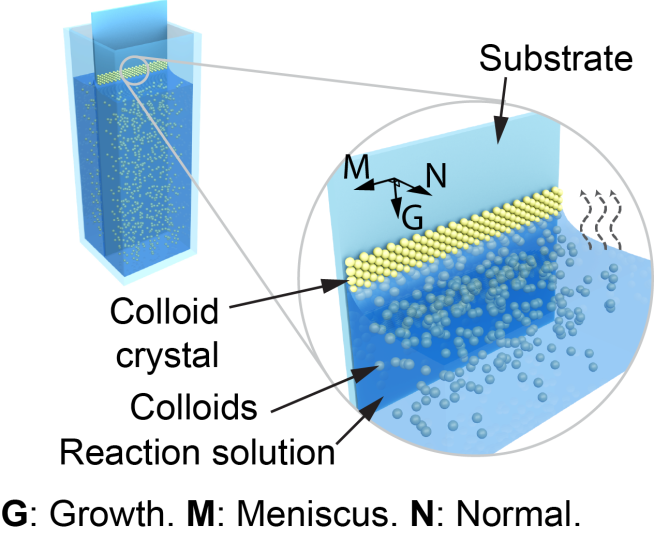
To do that, the team used a focused ion beam at the Harvard Center for Nanoscale Systems (CNS) to build a 3D image of the crystal layer by layer. This revealed defects in the crystal similar to defects found in naturally occurring atomic crystals like gold and copper. These defects, also known as dislocations, appear at the region where the colloidal particles rotated to the stable growth orientation.
Next, the researchers had to find what caused the dislocations and whether or not the dislocations themselves directly led to changes in particle orientation.
To answer that question, the researchers combined theoretical analysis with their observations of colloidal crystal growth.
"We noticed that during the self-assembly process, the particles on the surface of the substrate are still partially wet," said Li. "When the solution evaporates on a rigid substrate, the crystal dries and shrinks in such a way that leads to mechanical stress and defects, much like when wet paint dries it shrinks and sometimes cracks. When the crystal shrinks, the mechanical stress induces dislocations."
Using this analysis and observations, the researchers built a model to reproduce the self-assembly process. When they introduced the mechanical stress into the system, they observed a rotation in the orientations of the colloidal particles. When there was no mechanical stress, there was no rotation.
"Using theory, modeling, and observation, we answered this fundamental question about colloidal crystal growth," said Frans Spaepen, the John C. and Helen F. Franklin Professor of Applied Physics at SEAS and co-author of the paper. "Since mechanical stresses are often present in evaporating systems, we expect that the mechanical stress-induced dislocations and the corresponding rotation of the crystal particles revealed here are a universal principle for preferred growth in evaporation-driven colloidal assemblies."
"By taking advantage of the preferred growth and directional cracking, we can precisely control the crack pattern formation in evaporation-induced colloidal crystals and engineer crystals with different properties for different applications," said Aizenberg.
"This research also provides new insights into the growth patterns of synthetic atomic crystals and natural biominerals, such as eggs and shells," said Michael Brenner, the Michael F. Cronin Professor of Applied Mathematics and Applied Physics and Professor of Physics at SEAS and co-author of the paper.
The study represents the latest pioneering colloidal research to emerge from Harvard MRSEC. Brenner, Aizenberg and Spaepen are among a group of interdisciplinary and collaborative faculty at the Center who are working to create new classes of functional materials and unravel complex phenomena in soft materials with the goal of developing technologies to benefit society.
"As this fundamental research on soft matter demonstrates, our MRSEC fosters a highly collaborative culture of scientific discovery with real-world impact," said Jennifer Lewis, MRSEC Director and the Wyss Professor of Biologically Inspired Engineering at SEAS.
The research was co-authored by Carl Goodrich, Haizhao Yang, Katherine R. Phillips, Zian Jia, Hongshun Chen, Lifeng Wang, Jinjin Zhong, Anhua Liu, Jianfeng Lu and Jianwei Shuai.
The research was supported by the NSF’s Materials Research Science and Engineering Center under grants DMR-1420570 and DMR-2011754.